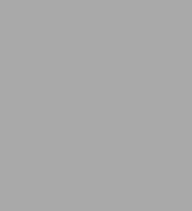
Discovery of the Life-Organizing Principle: In Search of the Fundamental Laws of Life
248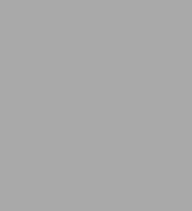
Discovery of the Life-Organizing Principle: In Search of the Fundamental Laws of Life
248eBook
Related collections and offers
Overview
Everything you wanted to know about physics, biology, and the world at large becomes straightforward once you understand the life-organizing principle. Author E.M. Elsheikh, a mathematician and longtime professor, examines what the principle tells us about nature and life in this academic work. He proves that the secrets of our world reside in the quantum information contained in our DNA and genome.
Discover the inner workings of life, and develop a better understanding of a maximum-action principle that explains self-organization, self-replication, and self-evolution. He also explores such topics as laws that describe phylogenetic evolution and ontogenetic development; little-known facts about genetics and evolution, including why Darwinian theory facilitates a more dynamic conception of human nature; extensions of quantum theory; and new foundations of knowledge.
By challenging the notions of mainstream biology and physics and questioning assumptions about life being a physical rather than a supernatural phenomenon, you’ll stumble upon truths that few others know. Get ready to go on a fascinating journey that challenges paradigms and leads you to the Discovery of the Life-Organizing Principle.
Product Details
ISBN-13: | 9781491727188 |
---|---|
Publisher: | iUniverse, Incorporated |
Publication date: | 03/20/2014 |
Sold by: | Barnes & Noble |
Format: | eBook |
Pages: | 248 |
File size: | 2 MB |
Read an Excerpt
DISCOVERY OF THE LIFE-ORGANIZING PRINCIPLE
In Search of the Fundamental Laws of Life
By E. M. ELSHEIK
iUniverse LLC
Copyright © 2014 E. M. ElsheikAll rights reserved.
ISBN: 978-1-4917-2717-1
CHAPTER 1
WHAT IS LIFE?
1.1—CHARACTERISTICS OF LIFE
Physicochemical Bases
During the nineteenth century, both chemists and biologists had been able to discover the elementary constituent of their subject matter. Biologists showed that the cell is the elementary constituent from which an organism is built and that all cells arise from preexisting cells. Chemists first focused their attention on inorganic matter and found that it consists of a small number of different atoms in definite proportions. However, when they came to analyze organic substances, the picture seemed quite different. Two substances might have exactly the same composition yet show distinctly different properties. For example, ethyl alcohol is composed of two carbon atoms, one oxygen atom, and six hydrogen atoms; so is dimethyl ether, yet one is liquid at room temperature while the other is a gas. Moreover, organic molecules contain many more atoms than the simple inorganic ones, and there seemed to be no reason the way they were combined.
Thus J. J. Berzelius (1779-1847) and other chemists decided that the chemistry of life was something apart that obeyed its own set of subtle rules and that only living tissue could make an organic compound. However, in 1828, German chemist Friedrich Wohler, a student of Berzelius's, produced an organic substance in the laboratory by heating ammonium cyanate compound, which was then generally considered inorganic. This spectacular discovery paved the way for all the miracles that biochemistry and (later on) molecular biology have been witnessing since then. Biochemists now have at their disposal all sorts of synthetics: explosived, insecticides, plastics, fibers, rubbers, and proteins.
Living matter contains elements, inorganic and organic compounds. The most abundant elements found in protoplasm are oxygen, carbon, hydrogen, and nitrogen. Other elements found in small quantities are calcium, phosphorus, chlorine, sulfur, potassium, sodium, magnesium, and iron. Some other elements not generally present that may occur in small quantities are lithium, boron, fluorine, aluminum, silicon, vanadium, manganese, cobalt, copper, zinc, selenium, bromine, molybdenum, cadmium, iodine, and barium. The nonmetallic elements oxygen, carbon, hydrogen and nitrogen are the most abundant in living matter because of their contributions to the formation of organic molecules. The metallic elements commonly constitute only a small fraction of the cell volume but are essential to many phases of cell metabolism.
There are no inorganic compounds in protoplasm that do not occur in the same form in nonliving matter. The most abundant of these is water, which constitutes 75 percent of living matter. Carbon dioxide is present as a dissolved gas. Also dissolved in the water of cells are salts, acids, and bases. Carbon dioxide is utilized by green plants in food synthesis and released in all cells as an end product of food breakdown. Many inorganic compounds in living matter are common salts, acids, or bases. When dissolved in water, their molecules tend to dissociate into ions, positively charged cations from hydrogen and the metallic elements and negatively charged anions from the nonmetallic elements. Table salt, for example, ionizes into sodium ions that are positive (Na+) and chlorine ions that are negative (CI-). Acids invariably form hydrogen ions (H+) and anions that differ with the kind of acid. Bases yield various types of cations and invariably hydroxyl ions (OH-).
A great many complex organic compounds occur in living matter: carbohydrates, lipids, proteins, and nucleic acids. These organic molecules are composed of a relatively small number of kinds of molecular subunits. The large molecules break down into their component units on hydrolysis (digestion), and synthesis occur through the reverse process of bonding by dehydration (condensation). Sugars and the products of their condensations (polysaccharides) are carbohydrates. A sugar consists of a carbon chain generally arranged in a ring structure, flanked by hydrogen atoms and hydroxyl (OH-) groups, the hydrogen and oxygen being present typically in the same proportions as in water. Many carbohydrates are hexose (6—carbon) sugars or yield hexose when digested. Pentose (5—carbon) sugars occur in nucleic acids and certain polysaccharides and are important in photosynthesis. The initial carbohydrate manufacture takes place in organisms in the process of photosynthesis. The energy stored, which comes from the sun, is then available for biological work through the oxidation of the carbohydrates that are produced. The carbohydrates are classified as monosaccharides, disaccharides, and polysaccharides. Monosaccharides are simple sugars like glucose (C6 H12 O6), one of the key molecules in cellular respiration, and ribose (C5 H10 O5) a subunit of nucleotides, which form nucleic acids.
Disaccharides, double sugars, consist of two linked monosaccharides. Examples: sucrose (C12 H22 O11), table sugar, and maltose (C12 H22 O11), derived in the digestion of starch. Sucrose is formed of one molecule each of glucose and fructose; maltose is formed of two molecules of glucose. Polysaccharides are formed from numerous linked monosaccharides. Example: starch, cellulose, and glycogen all have the same empirical formula (C6 H10 O5)x.
The lipids are chemically diverse molecules that are generally insoluble or only slightly soluble in water but are soluble in ethyl alcohol, ether, and some other organic solvent. Three types of lipids commonly occur in protoplasm:
Fats (true fats or neutral fats) are compounds that consist of one molecule of glycerol and three molecules of fatty acids. Each fatty acid consists of a long hydrocarbon chain that is saturated if the carbons contain the maximum possible number of attached hydrogen (as in palmitic acid) and unsaturated if any carbon atoms are double bonded, resulting in less hydrogen per carnon (as in oleic acid). Fats occur primarily as food reserve molecules, formed when food material is abundant and degraded when food is in demand.
Phospholipids are similar to the true fats, but one of the fatty acids is replaced by a phosphate group, usually with additional water-soluble molecules.
Steroids are structurally different from true fats, having four fused carbon rings with an attached carbon chain of varying length. In small concentrations, various forms of these compounds exert biological regulatory effects on sexual development and function as well as on certain aspects of metabolism in higher organisms. Common examples include cholesterol, vitamin D, sex hormones.
Proteins:
These are large molecules consisting of long unbranched chains of amino acids. Although the twenty or so different types of amino acids have distinctive structural characteristics and therefore distinctive chemical properties, they all have the same configuration at one location. This configuration, an amino group (–NH2) and an acidic carboxyl group (-COOH) attached to the same carbon atom, is the key to the manner in which adjacent amino acids are linked by peptide bonds. These bonds are formed between the amino group of one amino acid and the carboxyl group of the next, one molecule of water being removed in the formation of each bond. The remaining components of each amino acid extend from the protein as side chains (arms) consisting of hydrogen (as in glycine, the simplest amino acid) or of a variety of straight chain or ring compounds, which may also contain sulfur or additional oxygen and nitrogen.
Almost all cellular differences within an organism and among organisms can be traced to protein differences. Proteins are essential in the formation and maintenance of the structural and functional machinery of cells. They are after deamination (removal of –NH2), a source of energy in the diet being oxidized much as carbohydrates are. There are different types of proteins:
i. Proteins that function in structural capacity (e.g., keratins of fingernails, skin, and hair as well as collagens of connective tissue)
ii. Proteins that are hormones, regulators of metabolic processes (e.g., insulin)
iii. Proteins that are components of chromosomes (e.g., histones)
iv. Proteins that function in the transport of oxygen (e.g., hemoglobin)
v. Proteins that are organic catalysts (enzymes) controlling reaction occurrence and rate (e.g., enzymes of digestion and enzymes of cellular metabolism). Like other catalysts, they activate a reaction, but they are not used up in the process.
Nucleic Acids:
Nucleic acids are organic compounds that are even larger than proteins. As with proteins, they are composed of repeating subunits. In nucleic acids, these subunits are nucleotides. Each nucleotide consists of a nitrogen-containing organic base (chemically a purine or purimidine) a pentose (5—carbon) sugar (ribose or deoxyribose), and phosphoric acid. There are two types of nucleic acids: DNA (deoxyribonucleic acid), which has deoxyribose in the nucleotides, and RNA (ribonucleic acid), which has ribose as a nucleotide component. Each type of nucleic acid has four possible nucleotides, depending on which nitrogenous base is present. DNA nucleotides contain the purines guanine and adenine and the pyrimidines cytocine and thymine. The same kinds of nucleotides compose RNA, except that thymine is replaced by uracil. The sugar of each nucleotide is attached to the adjacent nucleotide through the phosphate, and the purine and pyrimidine are side branches.
The DNA molecule consists of two parallel nucleotide chains in a double helix, with the organic bases extending toward each other. These bases pair adenine only with thymine, and guanine only with cytosine, with stabilizing bonds (hydrogen bonds) holding the adjacent polynucleotide chains in position. As a result, the strands are complementary but not identical. DNA is predominantly found in chromosomes, whereby it performs basic functions in the control of cell expression and in the transmission of heredity information from one cell to the next cell generation. The theory that the pair of complementary polynucleotides that composes the DNA molecule separates, forming new DNA molecules after the synthesis of new complementary units, gives a chemical explanation of gene replication. In contrast to DNA, most RNA is found as single strand on a helix. There are three types of RNA found within the cell: ribosomal RNA, transfer RNA, and messenger RNA. In association with DNA, RNA controls protein synthesis.
Metabolism:
A basic characteristic of living systems is metabolism. The phenomena of metabolism include the taking in of various substances by the organism from the external environment, their assimilation and transformation, and elimination of breakdown products of decomposition. All these processes of transformation are attended by a multiplicity of various chemical, mechanical, thermal, and electrical phenomena and by a continuous transformation of energy. The potential energy of complex organic compounds is liberated through their breakdown and is transformed into heat, mechanical power, and electricity.
Synthetic energy demanding reaction of metabolism is called anabolism, and the reverse, breakdown, energy-releasing reaction is called catabolism. Metabolic reactions occurring within the cell require presence of enzymes. Enzymes are biological catalysts, compounds that accelerate chemical reactions, enabling the cell chemical machinery to operate under its relatively low temperature, low pressure, and relatively restricted PH range. An enzyme catalyzes a reaction by reducing the amount of energy necessary to activate it.
Metabolic reactions operate in sequence, the product of one reaction being the substrate of the next and each serving as an important link in the total chain of reactions. The metabolic sequences can be summarized under four categories: photosynthesis, respiration, biosynthesis, and digestion. Photosynthesis and biosynthesis are both anabolic reactions (more energy goes into the reaction than is released), whereas digestion and respiration, on the other hand, are catabolic reactions (releasing more energy than is required for the reaction).
Food production takes place in chlorophyll-containing cells by the process of photosynthesis. The energy involved is from light, and the raw materials used are water and carbon dioxide from the environment. The net effect, chemically, is reduction, leading to the formation of simple carbohydrates and accompanied by the release of free energy. Respiration is a complex series of intracellular reactions, releasing the energy stored in food. The chemical process is oxidation, and the net effect is the reverse of photosynthesis. The complete process requires oxygen; the original raw materials of photosynthesis, carbon dioxide, and water are given off as end products.
In biosynthesis, simple food molecules produced by photosynthesis or obtained in nutrition are combined (condensed) to form larger molecules. Large carbohydrate molecules (polysaccharides), representing stored food condensed from simple sugar. Stored fats of many different kinds are condensed from glycerol (derived from triose sugar) and fatty acids (long chain compounds derived from many combined acetyl groups). The production of the complex macromolecules of nucleic acids and proteins is controlled by the hereditary material. The cell usually manufactures a variety of large molecules through biosynthesis and assembles them into the structures of intracellular units. Thus the complex architecture of the cell is produced and maintained.
Because a cell membrane is impermeable to larger molecules, these larger molecules must be broken down (digested) for transport and or absorption through cell membranes. During the life of the cell, its various molecular components are continuously being built up and broken down. This gain and loss of molecules is dependent on the rates of synthesis and digestion.
Physicochemical bases of life are not only manifested in that living matter is composed of the same elements as nonliving matter but also certain principles basic to an understanding of physics and chemistry are important in biology. Thus, in both living and inanimate systems, energy may change from one form to another—from potential energy to the kinetic energy of heat or movement as well as from light energy to chemical (potential) energy.
Transformation of chemical energy into various forms of biological activity is accompanied by a change of part of the energy into heat, which is dissipated; such transfers are not 100 percent efficient. It follows that while no energy is lost in accordance with the first law of thermodynamics, there is a decrease in useable energy in the system in accordance with the second law of thermodynamics.
Bioinformation Generating Systems:
In the previous section, it was shown that biological systems, in the final analysis, do compose of the same element (atoms) that are found in inanimate systems and that the biophysical and biochemical transformations and reactions comply with physicochemical laws (i.e., they show no violations for these laws). In this section, we consider a process that is characteristically biological and has no counterpart in the inanimate world, namely the process of self-organization, which increases bio-complexity as manifested in the growth and evolution of biological systems. Ontogenetically as well as phylogenetically biological systems increase in biocomplexity, body size, or life span.
The cell is the basic unit of biocomplexity. Basic cellular processes such as cellular growth, cellular differentiation, and evolution are processes of increased biological organization and complexity. However, at this preliminary stage, let us consider DNA replication and protein synthesis, which substantiate the claim that DNA is a self-organizing and self-replicating system.
The complexity of proteins is unmistakable. For instance, the molecule of a blood protein called cerum albumin was found to contain the following amino acids (Asimov 1972): 15 glycines, 45 valines, 58 leucines, 9 isoleucines, 31 prolines, 33 phenylalanines, 18 tyrosines, 1 tryptophan, 22 serines, 27 thereonines, 32 cystines, 4 cesteines, 6 methionines, 25 arginines, 16 histidines, 58 lysines, 46 asartic acids, and 80 glutamic acids, a total of 526 amino acids of 18 different types built into a protein with molecular weight of about 69,000. All of these 526 amino acids are arranged in a definite order to constitute a definite structure. Clearly a cell cannot make such an arrangement randomly; there must be a deterministic process for generating this biocomplexity.
(Continues...)
Excerpted from DISCOVERY OF THE LIFE-ORGANIZING PRINCIPLE by E. M. ELSHEIK. Copyright © 2014 E. M. Elsheik. Excerpted by permission of iUniverse LLC.
All rights reserved. No part of this excerpt may be reproduced or reprinted without permission in writing from the publisher.
Excerpts are provided by Dial-A-Book Inc. solely for the personal use of visitors to this web site.
Table of Contents
Contents
Preface, ix,Acknowledgments, xiii,
Introduction, xv,
1 — What Is Life?, 1,
2 — The Problem with Evolution, 37,
3 — Paradigm Shift, 61,
4 — Quantum Information Fractal Field Theory (QIFFT), 75,
5 — Maximum Action Principle, 97,
6 — Evolution, 109,
7 — Growth and Development, 127,
8 — Ecosystem Dynamics, 139,
9 — Toward a Theoretical Biology, 151,
Conclusion, 193,
Appendix, 199,
References, 203,